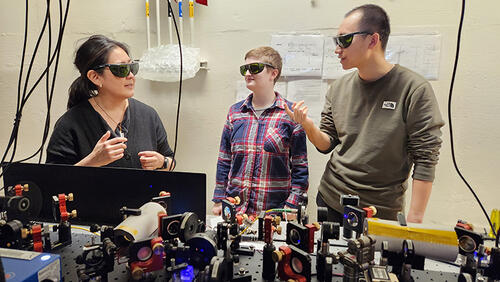
In honor of World Quantum Day (April 14), Wright Lab celebrates the following efforts by our scientists to explore the applications of quantum science and sensing to tests of fundamental physics.
Search for axion dark matter at Yale
Three experiments located at Wright Lab are searching for axion dark matter using quantum technologies. The experiments include: HAYSTAC (Haloscope At Yale Sensitive To Axion CDM), ALPHA (Axion Longitudinal Plasma Haloscope), and RAY (Rydberg Atoms at Yale ).
HAYSTAC is a tunable radiofrequency cavity resonator that uses an innovative quantum noise squeezing technique to speed up the data-taking of the experiment.
ALPHA will build on the success of HAYSTAC and search for even higher mass axions by employing a novel axion detector called a plasma haloscope; pushing the boundaries of quantum detection and microwave technology.
To extend the mass range accessible by axion dark matter search experiments, the RAY group is developing a single-photon detector for haloscope experiments, such as HAYSTAC and ALPHA, based on microwave transitions between highly excited Rydberg states in potassium atoms.
Wright Lab faculty Reina Maruyama, Keith Baker, Charles Brown, Karsten Heeger, and Steve Lamoreaux are involved in leading the axion dark matter search efforts.
Lamoreaux is also the co-author, along with Robert Golub, professor of physics at North Carolina State University, of “The Historical and Physical Foundations of Quantum Mechanics”.
Quantum science & computing in high energy physics
Keith Baker, D. Allan Bromley Professor of Physics, studies quantum information science in high energy physics, quantum entanglement, Bell’s inequality, and entanglement entropy. The Baker group demonstrates applications of machine learning, quantum computing, and quantum algorithms in physics analyses at high energies to better understand certain anomalies in data from high energy particle physics experiments. Baker is also the academic editor and co-author of “Quantum Entanglement in High Energy Physics”.
Search for dark energy with quantum sensors
Assistant Professor Laura Newburgh is part of Simons Observatory and CMB-S4, which use sensors that sit on the transition between the superconducting and normal-metal states (‘transition edge sensors’) to sensitively detect photons from the Cosmic Microwave Background. They are read out with superconducting quantum interference devices (SQUIDs), using new wide-bandwidth readout crates to many more sensors in a single connection than was possible before.
Single phonon detection using quantum acoustics
Professor Jack Harris is developing new technologies to control and study massive objects using light, and has been among the pioneers of the emerging field of quantum optomechanics. These quantum optomechanical sensors can detect tiny excitations of quantized sound (phonons). The Harris group is exploring superfluid helium as a medium for use in ultraprecise and quantum-enabled sensors in table-top experiments. One experiment uses quantum interactions to produce phonons that are particularly well suited to test the Standard Model of physics and to search for dark matter. A second experiment uses magnetic levitation to suspend a millimeter-scale drop of helium in a vacuum to study the quantum features in the drop’s motion.
Probing the nature of neutrinos, dark matter, and gravity
Associate professor David Moore develops new technologies and techniques aimed at answering some of the major outstanding questions in nuclear and particle physics about neutrinos, dark matter, the preference for matter over antimatter in the Universe, and the nature of gravitational interactions among quantum systems.
Moore is collaborating on the MAST-QG (MAcroscopic Superpositions Towards witnessing the Quantum nature of Gravity) experiment to test whether gravity has a quantum nature by levitating tiny diamonds in a vacuum to see if they become entangled through their gravitational interaction. The Moore group is using their expertise in precisely trapping nanoparticles in a vacuum to study the electromagnetic interactions between nanodiamonds.
SIMPLE (Search for new Interactions in a Microsphere Precision Levitation Experiment) is a small-scale tabletop experiment at Wright Lab that is used to study interactions involving neutrinos; to test gravity; and to search for dark matter, quantum phenomenon, and new forces. SIMPLE uses a technique called optical tweezers, in which a laser optically levitates, controls, and measures micron-sized spheres (microspheres). By measuring the motion of the microsphere, the group can precisely detect extremely tiny impulses, which are smaller than one-quadrillionth of the momentum transferred by a feather landing on your shoulder. The Moore group has developed the world’s most sensitive micron-sized force sensors, using them to search for dark matter interactions with the microspheres.
QuIPS is a new experiment located at Wright Lab that extends the techniques used by SIMPLE to even smaller nanoparticles and will measure the momentum kick from a single nucleus decaying within a particle. QuIPS will enable new searches for otherwise invisible particles emitted in nuclear decays, such as sterile neutrinos.
Connecting theory and experiment with effective field theory
Assistant professor Ian Moult’s research focuses on developing new techniques in quantum field theory for describing high-energy particle physics experiments, ranging from dark matter detection to elementary particle and heavy ion collisions at the Large Hadron Collider (LHC). A common theme in his work is the use of effective field theories, which allow calculations relevant for complex, real world experiments to be reduced to simpler, universal problems in quantum field theory.